MRI – magnetic resonance imaging – is a powerful medical technology that has revolutionized diagnosis of a very wide range of illnesses and injuries, greatly reducing or in many cases eliminating the need for exploratory surgery. It provides medical practitioners with two- and three-dimensional images, as well as high-accuracy cross-section, of internal structures and organs within a patient’s body.
Underpinning the results achieved by MRI scanning is a wide range of advanced technologies, including precision measurement techniques: the almost unbelievable sharpness of the pictures that MRI produces depends directly on measurements of basic electrical parameters.
MRI frequently sits alongside – and in some ways is complementary to computed tomography (CT). CT scans are based on X-rays and are best at imaging high-density structures (such as bones), whereas MRI scanning reveals the details of soft-tissue structures.
The working principle of MRI is based on nuclear magnetic resonance. In fact, what MRI actually detects is the magnetic resonance of the protons of hydrogen atoms contained in the water within the human body that represents up to 70% of body weight. In more exact terms, MRI observes the response of the hydrogen nuclei exposed to excitation by both magnetic and electromagnetic fields.
The collected energy per volume element (voxel) depends on the water distribution in the place under analysis, so, MRI can provide a three-dimensional image of the water distribution inside the human body. As each type of body tissue has a characteristic proportion of water within it, it becomes possible to image those tissues, and any deterioration, by looking at changes in water distribution.
Working Principle
To start, there is a need to know the working principles of the nuclear magnetic resonance (NMR). The nuclei of atoms have the property of behaving like magnetic dipoles or magnets when excited by a magnetic field (Figure 1). Nuclei of atoms have a spin – or magnetic moment – which we conventionally represent by a vector along the rotation axis.
In the absence of any external influence, this tiny magnet is not oriented in any particular direction. As soon as this magnet is illuminated by a constant and homogeneous static magnetic field (referred to as H0) it aligns with H0 in two directions: parallel and anti-parallel to the field. The nuclear magnetic moment is tiny and requires an intense applied field to achieve the alignment; the related magnetic induction B0 is commonly between 0.2Tesla and 3Tesla. In the following simplified explanation, only the parallel alignment is considered.
The alignment process is more subtle than a simple setting of the spin axis along the field lines. If we take the Z-axis (see Figure 3) as parallel to the applied field, the spin precesses or rotates around the Z-axis along a cone at angular speed ω0. The related frequency is called the Larmor frequency:
ω0=yB0
The precession speed is therefore proportional to the static magnetic field; for example, a field of B0=1Tesla gives a frequency f0 = 42.5MHz.
Resonance of the Nuclei
In order to observe the resonance of the nuclei, some energy has to be provided allowing nuclei to move from steady state to excited one. This is achieved by applying a high frequency magnetic field H1. When the frequency of H1 equals the Larmor frequency, resonance occurs and the nuclei move to a higher energy state.
During the application of H1, the spin axes of the nuclei are no longer aligned with H0 (Z-axis) but move into the X-Y plane. After the H1 excitation is turned off, the spin axes once again align with H0, and the extra energy they gained from the H1 excitation radiates away in the form of a damped electromagnetic wave (also known as relaxation). An antenna detects damped wave, yielding an induced voltage called free induction decay (FID).
It is the FID signal that the MRI’s computer processes to a 3D or section image.
Applying the Magnetic Fields
The static magnetic field H0, as previously noted, must be very intense, with very high stability and homogeneity within the volume inside the aperture of the MRI scanner, where the patient lies.
Most of today’s MRIs generate the static field by means of a superconducting magnet located around the cylinder of the scanner. The coils of the magnet are made up of niobium-titanium (NbTi) wires immersed in liquid helium at a temperature of 4°K.
The gradient coils superimpose a magnetic gradient to H0 in order to provide a spatial coding of the image. Imaging takes place in just one plane or slice at a time, and to ensure that signals are received only from nuclei in that plane, only those nuclei have to be pushed to resonance.
The appearance of the resonance is strongly dependent on the value of the magnetic field H0: The gradient coils superimpose a magnetic field to ensure that the final magnetic field is exactly equal to H0 only in the plane of interest.
How Gradient Coils Work
To create a gradient along an axis, a pair of coils is needed. In each pair, currents flow in opposite directions (the principle is shown in Figure 6).
In fact, three pairs of gradient coils are located around the cylinder of the MRI apparatus to create three orthogonal magnetic fields. Therefore, it is possible to adjust the magnetic field at any point in the volume of the cylinder. Gradient amplifiers operating in a closed servo-type loop drive the currents in the gradient coils (Figure 7). Each MRI therefore needs three such current control loops.
As can be seen from the principle of MRI outlined above, the quality, the clarity and the resolution of the images are directly linked to those of the magnetic field applied, and therefore to those of the current injected into the gradient coils. One of the key elements in the current control loop is the global accuracy of the current transducer.
In particular, the following parameters of the current transducer are critical:
- Extremely low non linearity error (<3ppm of measuring range)
- Very low random noise (low frequency noise from 0.1Hz to 1kHz)
- Very low offset and sensitivity drifts over temperature range (<0.3ppm/°K)
- Very high stability of offset versus time (one reason for this is the duration of MRI scans, some of which may last tens of minutes)
- Measuring range (around 1,000A peak)
- Bandwidth (–3dB point of 200kHz)
To reach these performance levels, Hall Effect current transducers – which were used in previous generations of MRI scanners – are no longer adequate. The solution developed by LEM, primarily for this application area, has similarities to the Hall Effect technique but offers significant advantages. It is described as a double fluxgate closed loop transducer and identified as type ITL 900. Although fluxgate technology has been available for some time, LEM was able to take this technology, adapt it, and improve it.
As well as precise current control in gradient amplifiers for medical imaging, the ITL 900 is equally applicable to measuring feedback in precision current regulated power supplies, current measurement for power analysis, calibration equipment for test benches, and laboratory and metrology equipment, which also require high accuracy.
In its present form, the technology is limited to a relatively narrow operating temperature (typically +10°C to +50°C). However, LEM officials are confident that the technology can be developed further and that the ITL 900 transducer could prove to be as significant for the future of MRI scanning as the Hall Effect transducers were for its introduction.
LEM USA Inc.
Milwaukee, Wis.
www.lem.com
About the author: Claude Gudel, is a research & development senior engineer with LEM USA Inc.
For more information, please contact Erik Lange, marketing & applications engineer for LEM USA Inc. at ela@lem.com.
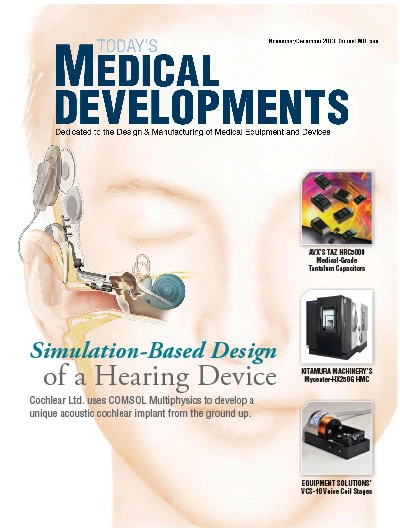
Explore the November December 2013 Issue
Check out more from this issue and find your next story to read.
Latest from Today's Medical Developments
- Machine Solutions acquires Alpine Laser LLC
- OSG USA’s PHOENIX PFDC indexable face mill cutter & inserts
- IMTS 2024 Booth Tour: Fagor Automation Corp.
- How Robotics and Automation are Transforming Manufacturing
- Quasar Medical acquires Ridgeback Technologies
- SMW Autoblok's TMS-2G quick-change system
- IMTS 2024 Booth Tour: Marubeni Citizen-Cincom, Inc.
- Star Cutter incorporates TRU TECH brand into grinding technology portfolio