![]() |
As medical device manufacturers increasingly explore the potential of biomaterials for their emerging device projects, engineers should consider the new level of scientific sophistication and increasing clinical applications of these materials for implant solutions. After decades of successful use and improved manufacturing techniques, now is the time to explore the full range of possibilities that textiles offer. Textiles can help device OEMs reach physicians as well, as they continue to embrace a more fibrous approach to solutions for repairing, replacing, and even re-growing integral cells for orthopedic, cardiovascular, bariatric, and other surgical indications.
Fibers as Foundation
From nylon to PLLA, the fibers currently used to create permanent and absorbable textile structures are as varied in performance capabilities as they are in profile. UHMWpolyethylene, polyester, polypropylene, polyetrafluoroethylene, and polyether ether ketone (PEEK) are just a few of the polymers popular for device components that require permanent implantation. Engineers are also increasingly looking to absorbable fibers such as PGA, PLLA, PDO, and other copolymers to aid in repair and growth before the body absorbs them. Correctly selecting the most effective polymer is critical to device performance, and developers must consider not only filament size and count, but also other characteristics. Those to consider are denier (linear density, which also includes the filament count in a multifilament fiber bundle and can have an effect on density and the resulting possibilities for processing); tenacity (the strength per denier); elongation (the fiber’s tendency to stretch or maintain its shape over time); and any post-processing – such as heat shrink (the amount of shrinkage at a certain time and temperature and determinative of possibilities for further downstream processing, including sterilization).
Range of Applications
Specialized textile engineering techniques enable contract manufacturers to create biomedical structures using a multitude of fibers by taking advantage of the properties of each unique material through processes that magnify strength, texture, flexibility, and other characteristics. From braiding and knitting to weaving and non-wovens, dedicated medical textile manufacturing partners can help ensure the best process and polymer fit for any application.
Braiding
Braiding is completed by the intermingling of fibers, usually at least three, in a maypole fashion to interlace the strands in a specific pattern. Combining fibers is common (i.e., mixing polyester with an absorbable material for partial degradation over time), as are complex engineering alterations to produce a customized structure with specific measurement requirements. Braiding around mandrels to create hollow tubes or flat braiding is also possible, and because it enables precise dimensions and exact geometry, can be used for orthopedic applications such as ligament and tendon repair.
Braiding produces a structure with a high degree of strength, but without a large surface area. For small, inter-spinal cavities or joint spaces, where repair or replacement pieces for torn or floating tendon are needed, strength is necessary, but must be in a compact form. Applications especially suited to braiding include knee, shoulder, and small joint arthroscopic procedures, because strength, the ability to expand and compress, and other performance capabilities can be created according to the needs of the particular joint. Sutures designed for general surgical applications are also braids, designed to prevent repeat tears, and resist abrasion and wear over time once internal injuries have healed and normal strength of movement is restored.
For cardiovascular applications specifically, strength requirements are often more precise, especially for aortic and other repair graft sewing threads. Small diameter braids are particularly useful for these applications, thanks to their relative degree of strength in a thinner form factor. Braids also secure well with other surgical sewing applications, and can be made with the textile’s polymer itself or designed using another high-strength material.
![]() Braiding is completed by the intermingling of fibers, usually at least three, in a maypole fashion to interlace the strands in a specific pattern. |
Knitting
Multiple knitting techniques exist for developing biomedical textiles, and among them are warp knitting and weft knitting (the latter, like braiding, is also common for making tubes). Based on a series of organized loops hooked together via the warp or weft process, knitting involves more individual fibers than braiding and can produce a structure of greater intricacy and more precise performance capability. Thanks to the concentration of power provided by the interstitial spaces between fibers, knitted textiles deliver superior stretch without sacrificing strength or increasing thickness. Orthopedic meshes for indications such as lumbar and cervical disk devices are especially good fits for this technology, which brings a strong but supple element to devices that must accommodate movement both at the site of the defect and in the surrounding soft tissue areas where organ architecture and function are affected. Likewise, many cardiovascular applications such as valve repair patches and rings utilize flat knit fabrics and tubular conduits, because their defined apertures and conformability fit the low profile, high-strength requirements necessary for device function.
Orthopedic and bariatric applications ideally suited for knitted structures also include those that undergo more severe instances of movement and stretch, such as containment sleeves for spinal disk repair and stomach stapling, as well as implants and procedural assistance pieces for the knee, shoulder, and spine. Likewise, general surgery applications such as surgical meshes, urogynecologic slings, prolapse devices, and hernia repair devices are all made possible by the combination of strength and flexibility that comes from the knitting process.
Weaving
Weaving produces structures that consist of longitudinal fibers held together by perpendicular cross-fibers, which allows them to provide thickness and strength without the stretch of knits or braids. With high tenacity, they are lightweight and stable, but hold their shape for support, repair, and replacement functions that must retain their original form. Orthopedic applications that particularly benefit from these advantages are spinal restoration and tendon repair, which must delivery density within a confined space and maintain strength over time without turning loose or showing excessive creep with age.
Woven tapes and tubes can also be designed to meet specific requirements of strength, porosity, and geometry. Vascular grafts, for example, require strength, but must keep from bulging to maintain the integrity of the device. Architectures include monolayer, multilayer interlock, and tubular, with bifurcation or tapering to accommodate anatomic and deployment requirements. These techniques are most critical for woven grafts supporting endovascular stent systems designed to prevent abdominal aortic aneurysm rupture, as well as for other percutaneous coronary intervention stent procedures and peripheral vascular grafts throughout the extremities.
Weaving is possible in a variety of geometric styles and is used primarily to ensure low elongation and maneuverability, such as in implantable tethers that support weight-loss surgery and in urological and gastrointestinal catheters. Cardiovascular, bariatric, and other surgeons are increasingly interested in incorporating thin, durable fabrics that can be folded during surgery and then unfolded or otherwise manipulated without affecting the integrity of the device.
Non-Wovens
Traditionally, non-woven structures have seen their greatest use in tissue engineering applications and absorbable scaffolds, but these kinds of textiles are increasingly being incorporated into orthopedic and cardiovascular devices using fibrous components, such as suture fasteners and pledgets, as well as reconstruction procedures throughout the body. Usually composed of felt created by a carding and needle-punch process, non-woven structures provide greater surface area than most other textiles, as well as a unique 3D structure that helps to encourage cellular in-growth and proliferation for repair and replacement functions.
Non-woven felt properties depend upon the type of needle, number of punches per measured area (usually a square centimeter), and amount of fiber entanglement within layers. Of particular importance is absorption profile, which controls the lifespan of the structure as it is replaced by natural cells within the body and is precisely engineered to maintain material integrity as long as its support function is required. Most non-wovens are scaffolds composed of absorbable biomaterials, meant to facilitate regrowth before disintegration. Examples in orthopedic and cardiovascular indications include tubular conduits for vascular replacement technologies, heart valve repair, and orthopedic injury devices.
![]() Top: Specialized textile engineering techniques enable contract manufacturers to create biomedical structures using a multitude of fibers. Bottom: Weaving produces structures that consist of longitudinal fibers held together by perpendicular cross-fibers, which allows them to provide thickness and strength without the stretch of knits or braids. |
Fiber Prep, Secondary Processing
Once structures have been developed, creating finished textile components for device manufacturers often depends on additional processing techniques to take full advantage of the capabilities of each material. There are a multitude of manufacturing processes to aid in the creation of customized textiles that fit precise specifications of shape, size, and performance without sacrificing quality, including:
Sewing – The process of joining polymers together in a hem or multilayer system by connecting multiple finished textiles into a larger implant structure, sewing is especially important when building composite device components from dissimilar materials.
Cutting – Cutting enables specific dimensions for flat and molded structures, and includes die, laser, and ultrasonic techniques, as well as staple cutting. Staple cutting, which produces fibers of equal staple lengths, is especially beneficial for creating uniform scaffolds, where matching dimensions are critical to function.
Shaping – Shaping is used to mold textile structures into specific geometries for the most efficient implantation and in vivo performance. Shapes include everything from tubes and cones to disks and cylinders for medical devices.
Texturing – The process of adding crimp and bulk to otherwise straight materials in order to join them together, texturing is especially important to the creation of tissue scaffolds in orthopedic and cardiovascular regeneration applications.
Twisting/Plying – The process of twisting textile fabrics to increase their consistency, strength, and stability, twisting and plying help improve structures’ tenacity and resistance to abrasion with greater density.
Swaging – Like shaping, swaging helps mold structures into device components of particular dimensions by reducing the diameter of fibers and oftentimes connecting them together at their ends to create a different profile.
Tipping – The process of terminating the end of a fabric (most often a braid), tipping creates a rigid end that can be used to improve the handling of a braid or other fabric and can improve the pushability of a textile.
Biomaterial printing – Using medical-grade inks, contract manufacturers use state-of-the-art medical pad printing equipment to print directly onto scaffolds, meshes, and other finished textiles to aid in packaging, surgeon identification, and more.
From fiber selection to engineering and post-processing, using biomedical structures in medical device development requires a commitment to clinical performance and advanced textile expertise from start to finish. For device engineers, choosing the right partner is the key to fully exploiting the unique power of biomaterials for the next generation of device design.
Biomedical Structures
Warwick, R.I.
www.bmsri.com
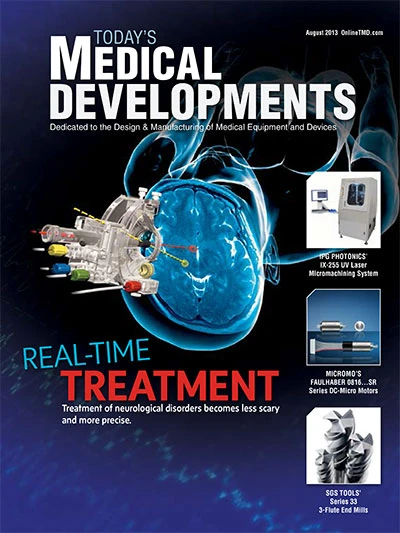
Explore the August 2013 Issue
Check out more from this issue and find your next story to read.
Latest from Today's Medical Developments
- Robot watched surgery videos then performed with skill of human doctor
- Master Bond’s EP21LSCL-2Med two component non-cytotoxic epoxy
- IMTS 2024 Booth Tour: Tornos Technologies
- A Primer on Defense Contract Manufacturing
- September 2024 USMTO orders up from August 2024; up from September 2023
- Sandvik Coromant's automatic tool change (ATC) for turning centers
- IMTS 2024 Booth Tour: Vollmer
- Supply chain vulnerabilities amid rising cyberattacks