
Developing parts and devices for the medical industry is a challenging, high-stakes process where time to market and accuracy are critical to success. Evaluating new product designs and materials; iterating quickly when needed; and selecting suppliers that can rapidly, consistently, and cost-effectively deliver prototypes and low-volume production, are important in attaining success.
What to look for in a manufacturer
The medical product development process is all about successfully speeding through the FDA gates, so a manufacturer that can quickly deliver quality prototype parts is crucial. One that has excess production capacity won’t be swamped by a large, rush order that could add unnecessary time to the process. A manufacturer that has successfully removed inefficiencies should be able to provide prototype parts in a few days, not months.
The manufacturer should be fast enough to allow multiple iterations in a condensed period, and have the scale to allow for multiple iterations at the same time, particularly for injection molding.

Competitively priced injection molding enables cost-effective short runs so product developers have more time to react with shorter lead times.
In addition to quick turnaround, look for a manufacturer that offers fast, automated quoting so you can get parts as soon as possible. Time is certainly saved when you can upload a 3D CAD model and receive an automated quote within a few hours.
Look for manufacturers that give immediate design feedback to improve the manufacturability of the parts when the quote is delivered.
Always use an experienced manufacturer that has a reputation and track record for fast, consistent, quality prototype parts on time. Also, can they execute what you ask every time? Do they have a mechanism to apply lessons learned further down the development stage? Do they have scale to do on-demand orders? Can they closely replicate end production manufacturing methods? Do they have materials used in the medical industry and a basic understanding of medical approval processes? If not, you may be using the wrong manufacturer.
Additive manufacturing
Often called 3D printing, additive manufacturing (AM) is an excellent way to quickly evaluate new product designs without making compromises due to complex part geometries and additional tooling costs. Design changes are relatively easy to make and low cost.
Disadvantages of 3D printing for prototyping are higher part costs; limited color and texture choices; and in certain instances, plastic-like materials will differ from the final production material used in processes such as molding and machining. If the surface finish, texture, color, and coefficient of friction vary from the end material, it is difficult to accurately assess the subtle needs and benefits of these properties.
The largest advantage 3D printing offers is accurate form and fit testing as the build process of additive technology can accurately produce the form and size of the desired part, making it very useful for early evaluation of new medical parts. Typically, a medical device developer will use 3D printing to identify design flaws, make changes, and then make second-generation machined parts or invest in tooling to create injection-molded parts.
Many materials can be 3D printed, however, regardless of the material used, the manufacturer of the finished good is responsible for ensuring parts and materials safety and efficacy in the application and environment in which they will be used.
Implantable parts and devices must meet a higher standard of biocompatibility, and all risks to the patient from the materials used need to be understood.
Stereolithography (SL), one of the more frequently employed additive technologies, is regularly used for producing models, prototypes, patterns, and, on occasion, production parts. The process involves building a part (or even a multi-part device as one) in single, thin layers by curing a photo-reactive resin with a UV laser or similar power source. SL is often used to create prototype parts in clear materials to view the flow of liquids through the parts. Some other processes may result in porosity or surface roughness that could impair any desired watertight properties. To aid this process, it’s important to select materials that resist water absorption and are formulated to be clear. For example, 3D Systems’ Accura ClearVue plastic is designed to produce near colorless parts for a range of applications.
For form and fit evaluation of devices, high-resolution stereolithography has expanded applications for medical devices/parts. Rapid prototyping using SL enables near effortless design iteration for form and fit. In addition to accelerating this process, 3D printing allows product designers to focus on the role the part needs to perform, relying on the part’s strength, or the shape and fit, with minimal reliance on the material’s properties.
Selective laser sintering (SLS) is an additive manufacturing process that uses a CO2 laser to draw onto a hot bed of thermoplastic powder. The laser lightly sinters – fuses – the powder into a solid. After each layer, a roller deposits a fresh layer of powder on top of the bed and the process repeats. SLS uses thermoplastic nylons, so parts are accurate and exhibit excellent toughness, however they have a rough surface and lack fine details. SLS can be used to create tough, durable medical prototypes.
Direct metal laser sintering (DMLS) is another additive manufacturing technique used in medical part and device prototyping. The process uses a laser to sinter powdered metal to form fully dense metal parts such as stainless steel and titanium components common in medical devices.
When selecting a 3D printing part supplier, look for an organization that has the reputation for accuracy, quality, speed, and reliability to ensure good quality parts as fast as possible.
CNC machining
CNC machining starts with a block of material before a tool removes material to create a part. This prototyping method often plays a significant role in the early and end-of-life stages of a product’s life cycle because there is no tooling expense and products can be supplied quickly and relatively inexpensively.
Machining enables those developing medical devices to test several different part designs in parallel, which in turn, shrinks their development cycle. This upfront, simultaneous testing of prototypes with the same product strength and density as the final product helps device developers be better prepared for passage through FDA gates.
Because device developers can’t merely pivot if they fail a gate – they need to start over – testing multiple design options at each gate offers a greater chance of success to emerge quickly at the end of the process with the right product.
Machining is also important for testing parts and devices with engineering-grade materials. Machined metals and plastics can give the same in-hand feel and weight as the final product, eliminating false information from doctor testing. For any metal prototyping, CNC machining is usually the best choice for testing the product’s strength and weight.
At the end of a device’s life, when demand begins to dwindle, CNC machining can play a cost-effective role to satisfy a need for only a small run of parts. On-demand production limits unnecessary warehousing space for excessive product and the associated financial risks.
Depending on the complexity of the part, CNC machining can be a cost-effective choice for production volumes less than 200. For more than 200 parts, or if design changes are possible before all testing is complete, creating aluminum tooling for injection molding makes sense versus CNC machining.
Injection molding
Injection molding is a common production method during medical part and device development, and can provide production parts made from plastic, metal, and liquid silicone rubber (LSR) materials as early as possible – an important benefit during the FDA gating process. This process offers repeatable, reliable, and consistent results. For most injection molding manufacturers, low-volume is approximately 10,000 parts.
The disadvantages of injection molding at low volumes are the upfront tool cost spread across a low number of parts and the risk that design changes may be necessary after a tool is made, which can be costly. However, using low-cost aluminum tooling versus expensive steel ($50,000 or more), can reduce the financial risk of the initial tooling investment.
It’s important to understand some common shortcomings of injection-molded parts. Some flash, parting lines, weld lines, knit lines, gate marks, and ejector pin marks typically are present on the final part. Extremely challenging or poorly designed injection-molded parts will likely cause challenges later. Parts designed to the best injection molded standards and practices will provide the largest operating window to produce good parts.
Materials
Device developers have many plastic, metal, and liquid silicone rubber materials in various grades available to them during prototyping and low-volume production, and each offers different properties and application.
Due to the sterilization need for medical devices, ensuring a material can withstand autoclave, E-beam, or gamma sterilization is important. Material biocompatibility is also critical in facilitating healthy interaction for devices that encounter patients. PEEK and PEI are two plastic materials with long-term biocompatibility that withstand steam autoclaving while keeping rugged physical properties.
USP Class VI and ISO 10993 tests are designed to assess the biological reactivity of various plastic materials in vivo, and while this testing is not a substitute for biocompatibility testing, it often is used by manufacturers to classify materials. Many plastics suppliers find it helpful to have their resins certified as USP Class VI and ISO 10993, especially if the resin is likely to be used in medical devices.
There are many metals that can be used to prototype parts via CNC machining and 3D printing processes as well as metal injection molding (MIM) for increased volumes. Proto Labs, for example, has more than 30 hard and soft metals that can be machined; a handful of available additive metals such as cobalt chrome, Inconel, and titanium; and stainless steel and nickel steel used for injection molding. Particularly hard, tough, and strong, 17-4 PH stainless steel is useful for medical instruments.
Liquid silicone rubber (LSR) is a regular material found in medical products due to its thermal, chemical, and electrical resistance; biocompatibility; and ability to retain its properties at extreme temperatures during sterilization. LSR molding is a common manufacturing process used to achieve quality LSR parts, which can be manufactured in low volumes in a few weeks.
Overmolding – molding a soft, flexible part on the outside or inside of a hard part – is growing in the medical industry and often is more cost effective than assembling multiple components. The over-molded material, such as an elastomer or a liquid silicone rubber, must be easy to clean and should be considered as one part in the design. A surgical device with a rubber over-molded handle is a good example.
There are advantages to using a clear material in prototyping to view the flow of liquids and gain a greater understanding of how everything works together. Parts may be less cosmetically appealing, but if it’s prototyping purpose is to monitor fluid interaction inside the part, cosmetics can be addressed in future iterations.
Design considerations
A method to track iterations that is repeatable and has a low risk of failure or human error should be developed for example, using different color-coded parts to track iterations. Note that major color changes can create molding issues, such as splays and knit lines that can vary by color.
End-use medical products need to be produced from a medically certified material and manufactured in an appropriate environment, such as a cleanroom for some parts. Due to a longer development cycle, it’s important to select a material that won’t be discontinued during the expected production life of the product, or a manufacturer will need to re-pass gates to prove a new material works. Choose materials that don’t have availability issues that could disrupt manufacturing schedules.
Improving probability of success
“It’s important to get the types of materials that will be used in the final product – and particularly materials that interact with the patient – as soon as possible in the testing phase,” says Paul Jossart, manufacturing manager at Nova-Tech Engineering. “The manufacturer must be able to make the device to meet the design intent so they know the device will perform satisfactorily later.”
The faster a manufacturer can get product prototypes into an engineer’s hands, the faster the device can get to market. Because the physics of materials vary, matching the characteristics of the final product material is important to get valid test results.
“Product design and process need to work together for successful product development,” Jossart says. “Hardly anyone ever gets it right the first time. I don’t know how you’d do it without rapid prototyping. The ability to find a prototyping supplier that can quickly turn low-volume parts is critical to success.”
Failing fast, learning what doesn’t work as soon as possible, then working to iterate quickly, is important to passing the FDA gates without having to go back and re-pass them. The right manufacturer can help you do that, moving your device one step closer to market launch.
Proto Labs
www.protolabs.com
Nova-Tech Engineering
www.ntew.com
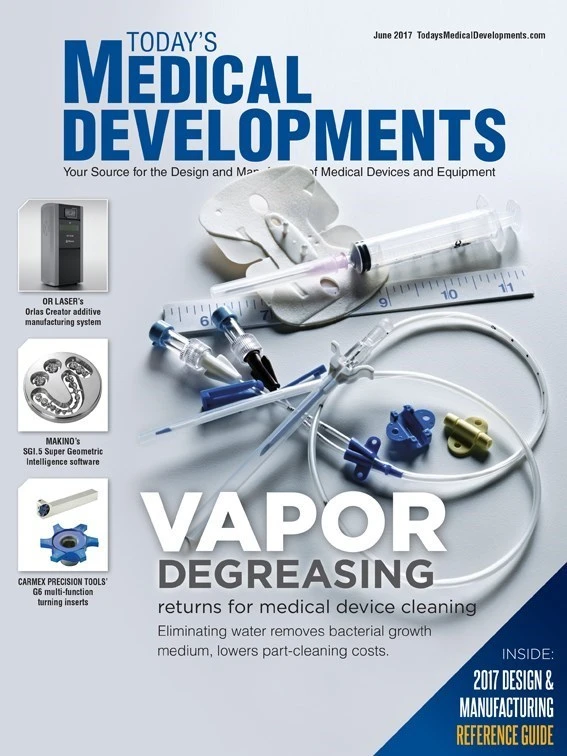
Explore the June 2017 Issue
Check out more from this issue and find your next story to read.
Latest from Today's Medical Developments
- Renishaw receives Industry Partner Award
- norelem's modular clamping systems for metrology
- IMTS 2024 Booth Tour: INDEX Corporation
- Robot watched surgery videos then performed with skill of human doctor
- Master Bond’s EP21LSCL-2Med two component non-cytotoxic epoxy
- IMTS 2024 Booth Tour: Tornos Technologies
- A Primer on Defense Contract Manufacturing
- September 2024 USMTO orders up from August 2024; up from September 2023