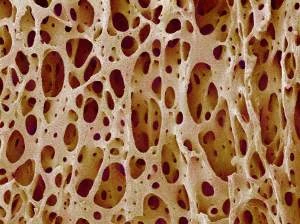
Innovation continues to be an important driver for profitability within the medical devices industry. Indeed, the largest portions of industry profits come from products introduced in recent years, due mainly to the short lifecycle of three to five years for new medical device products, compared with the 18 to 20 years associated with a new pharmaceutical drug.
Some of the most exciting innovations happening within the industry are in the area of materials and surface coatings. Materials and surface coatings have long needed to be biocompatible in order to be used in medical device applications, such as orthopedic and vascular implants, dental and drug delivery systems. Increasingly, however, more active material properties such as bioactive, biodegradable, biointegrative and controlled-release are becoming the innovation drivers in medical devices materials technology. There are high expectations that these new developments will aid patient recovery, reduce complications and eliminate the need for repeat procedures. Therefore, it is expected that these innovations will increase the long-term success rates of medical procedures, and improve the comparative cost-benefits analysis within the healthcare industry.
IS IT BIOCOMPATIBLE?
Biocompatible implies that the materials or devices employed provide the collective ability to perform a function for the host – the person in which the medical device is used – in a specific application, while not having side-effects on the biological system.
The use of biocompatible materials and coatings over the past 60 years is well documented for a range of different applications. For example, metals such as stainless steel and cobalt alloys have been widely used for orthopedic implants in joint prostheses applications because of their mechanical behaviors, including elastic modulus, wear and fatigue. Orthopedic implant devices are likely to have undergone some surface coating or modification to reduce the effects of corrosion on the body – for example, render them biocompatible – or to reduce the potential of mechanical failure.
Surface modification techniques are used extensively to make devices biocompatible. For example, the use of Parylene coating polymer is well known as a thin film surface coating for medical devices such as pacemakers; the surface coating acts as a chemical, moisture and electrical barrier, hence making the device more acceptable to the human body.
DOES IT DISSOLVE?
Biodegradable and bioabsorbable materials and devices used in a medical context are those that dissolve away into the body after a period of time, once their usefulness has reached an end.
Biodegradable polymers can be used in many medical applications. There are two main types of polymers used, natural-based or synthetic-based. The synthetic-based polymers have found many more uses as they are less likely to induce toxicity and infections. Furthermore, devices from synthetic polymers can also be produced with a higher predictability of mechanical and physical properties, such as tensile strength, elastic modulus and degradation rates.
Polymers belonging to the polyglicolic acid (PGA) and polylactic acid (PLA) families are being used in an increasing number of biomedical applications, such as sutures, surgical adhesives and drug delivery. For example, absorbable sutures manufactured from synthetic polymer material last long enough to allow the body to heal before breaking down and dissolving away within the tissues; therefore, no additional medical procedures are required. These are now used extensively for internal suturing applications.
There are two more advanced applications where biodegradable synthetic polymers are being used. First, they are being combined with a drug, or other active agent, for controlled release type applications. This involves the device being pre-designed with an active agent or drug that is released as the polymer degrades within the body. Second, they are also being considered for use as 3D scaffolding for tissue engineering applications in combination with bioactive substances such as calcium-phosphate, bioglass and bioceramic materials.
BIOACTIVE ABILITY
Bioactive means having an effect upon a living organism and, in a medical devices context, this can apply both to materials that are able to interact with living tissues or to a device from which an active agent or drug is released. In terms of materials, the most developed bioactive materials have been based on glass and glass-ceramic compounds including bioglass, apatite-wollastonite (A-W) glass-ceramic, and calcium phosphate materials for skeletal repair and reconstruction procedures.
The use of bioglass, glass-ceramics and calcium-phosphate as bioactive material for bone regeneration is well-documented. It is found it to have excellent biocompatibility and bonding capabilities with bone due to the similarity of their materials’ characteristics. The rate of bone formation and the strength and stability of the bond is dependent on chemical composition and microstructure of the bioactive material. Bone is known to be a natural nanocomposite, which consists of approximately 58% apatite, 12% water and 25% organic substances, principally collagen. The bioactive glass results in the formation of a biologically active hydroxyl-carbonate apatite (HCA) layer; this is chemically and structurally similar to the mineral phase in bone and provides direct bonding between the host’s soft connective tissues and the potential implant material.
A-W glass-ceramics developed over the same period have better mechanical properties than bioactive glass, such as bending strength, fracture toughness and Young’s Modulus. A-W glass-ceramics are finding useful applications in load-bearing situations, such as vertebral prostheses and iliac crest replacement.
More recently, calcium phosphate-based materials have found increasing use in a range of skeletal applications. The synthetic calcium phosphate ceramic hydroxyapatite (HA) and tri-calcium phosphate is widely used in dental, spinal fusion, cranio-maxillofacial reconstruction, and treatment of bone defects, fractures and joint replacement. Factors such as chemical compositions, phase purity and micro-structural porosity define the compounds’ compatibility with the local bone tissue, hence determining the success of the compound for new bone formation in a given application.
However, the mechanical properties of calcium phosphate ceramics are poor for load-bearing implant applications and cannot be used in isolation; for example, in joint replacement. For load-bearing implants, plasma-spraying of an HA coating has become a widely adopted method to improve the useful lifetime of the implant. The combined load-bearing capabilities of the base structure (normally a bioinert metallic), together with the accelerated bonding with surrounding tissues and enhancement of new bone formation (due to the HA compound) has lead to better outcomes for the host with a significant reduction in the need to repeat the replacement procedures.
Some of the most promising new areas for biodegradable and bioactive materials are now emerging for applications around tissue engineering. In this application, biodegradable polymers and bioactive glass and ceramics are being combined into composites materials to produce 3D scaffolding with high porosities suitable for tissue engineering. The ceramic particles add significantly to the degradable polymer, by adding strength and stiffness, and have the bioactive ability to adhere and encourage new bone growth for the implant. As the body regenerates, the tissue implant degrades and dissolves away within the body.
CONTROLLED RELEASE
The biodegradable polymers discussed earlier are attractive as potential drug delivery implants; once implanted they need not be retrieved or further manipulated as they simply degrade as a non-toxic by-product of the procedure. The degradation and erosion of the polymeric can also be used to control the release of drugs at a desirable rate. Research into controlled release has become more important as some of the more novel drugs being developed are more potent and less soluble then traditional drugs. The controlled drug released through implantable technologies offer three distinct advantages to traditional methods:
- They localize the delivery of the agent or drug at the site where the drug reaction is needed, reducing harmful effects to other parts of the body.
- They sustain drug delivery over a period of time without continuous interventions to the person undergoing treatment.
- They improve the in vivo stability of the agent or drug as the polymer used will protect the agent until it is released in the desired location within the host.
The active agent or drug is encapsulated inside microspheres created within the polymer material by controlling the porosity during manufacture. As the polymer degrades and erodes, the active agent or drug is released. The technology is now being implemented into numerous applications.
For example, coronary angioplasty with stenting is becoming increasingly popular as an alternative to coronary artery bypass grafting (CABG) for certain types of coronary artery disease. Angioplasty and stenting offer advantages to CABG as they can be conducted via keyhole surgery which results in faster recovery times. First generation devices were all metal in composition, and the procedure was prone to restenosis and further corrective intervention was often required (up to 40% of the time). Restenosis occurs when a treated vessel becomes blocked again after the initial procedure, due to the build-up of scar tissues. To overcome such issues in these new procedures, second generation devices are being introduced – drug eluting stents – that are reducing the incidence of restenosis, improving patient recovery.
Drug eluting stents contain specific drugs that are released within the body to prevent the build-up of scar tissue. The drugs are normally contained within a polymer coating applied to the stent, and control released near the implant to stop the build-up of scar tissue. The application has become more complicated from a materials and coatings prospective. Not only must the materials and coatings that are used meet mechanical and biocompatible requirements of the host’s body, but they must also focus on carrying and controlling the release of the drug or agent used to stop the build-up of scar tissue. Third generation devices could potentially be totally degradable and would leave the body completely after repairing the blocked vessel.
CONCLUSIONS
Some of the most exciting innovations within the medical device industry are in the area of materials and surface coatings. Increasingly more active material properties, such as bioactive, biodegradability, bio-integration and controlled release are becoming the innovation drivers in medical devices materials technology. There are high expectations that these new developments will aid patient recovery, reduce complications, and eliminate the need for repeat procedures within the healthcare industry.
CERAM
Staffordshire, United Kingdom
http://www.ceram.co.uk

Explore the July 2009 Issue
Check out more from this issue and find your next story to read.
Latest from Today's Medical Developments
- Flexxbotics’ Guide to Robotic Machine Tending Projects
- What will 2025 look like in the manufacturing industry?
- TriMech is selling, supporting One Click Metal's BOLDSERIES system
- Keep up with the latest in additive manufacturing through our free webinar
- How Vision Measurement and Inspection Ensures Maximum Quality Control
- Sir David McMurtry, Renishaw’s co-founder, passes
- Weiss-Aug Group expansion in Pennsylvania
- Siemens’ MACHINUM machine tool digitalization software portfolio